Scanning Electron Microscopy (SEM) is a ground-breaking imaging method that enables scientists and researchers to investigate the delicate features of the microscopic world with unparalleled precision. SEM produces high-resolution pictures of the surface topography and composition of diverse materials by using a focussed beam of electrons. This blog article attempts to provide a thorough explanation of SEM, including its history, concepts, sample preparation techniques, imaging process, instrumentation, and an overview of its diverse applications.
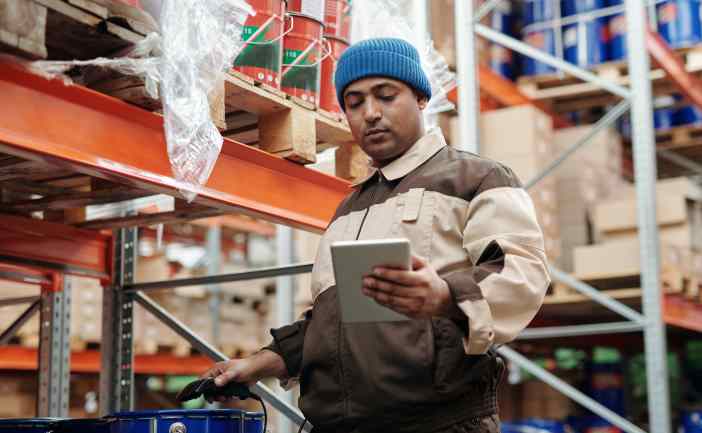
History of invention of Scanning Electron Microscopy (SEM)
- Ernst Ruska proposed the notion of electron microscopy in 1931, which led to the development of the first transmission electron microscope (TEM).
- The Beginnings of SEM: Manfred von Ardenne introduced the first SEM in 1937, however it was primitive in comparison to modern systems.
- Technological Advancements: SEM made significant progress in the 1960s and 1970s, with the development of scanning coils, improved electron optics, and signal detection systems.
- Modern SEM: Technological advances in computer technology and electron optics have resulted in the creation of sophisticated SEM systems with higher resolution and enhanced imaging capabilities.
Scanning Electron Microscopy (SEM) Principles
- SEM makes use of an electron beam generated by an electron source, which can be a tungsten filament, a field emission gun (FEG), or cold field emission.
- Electron-Matter Interactions: When an electron beam interacts with a material, it generates a variety of signals, including secondary electrons and backscattered electrons.
- Scanning and imaging: A raster pattern of electron beams scans the sample surface, and the collected signals are used to create images with detailed surface topography and compositional information.
Preparation of the Sample for Scanning Electron Microscopy
- Fixation and Dehydration: To retain the structure of biological samples, fixation with fixatives such as glutaraldehyde is followed by dehydration with a series of alcohol solutions.
- Coating: To minimise charge accumulation and increase image quality, non-conductive samples are coated with a thin coating of conductive material (e.g., gold or carbon).
- Mounting: To assist handling and positioning within the SEM chamber, samples are attached onto holders or stubs with adhesives or conductive tapes.
Preparation of the Biological Sample
- Fixation: Fixatives such as glutaraldehyde are used to retain the cellular structure of biological materials and prevent deterioration.
- Dehydration: To reduce water content without causing structural damage, samples are dehydrated using a series of alcohol solutions.
- Critical Point Drying: Critical point drying using liquid carbon dioxide is used to prevent shrinkage and collapse of fragile biological samples.
- Coating: To allow electron conduction, non-conductive biological samples are often coated with a conductive substance such as gold or platinum.
Scanning Techniques and Image Formation
- Beam Scanning: Using scanning coils controlled by the SEM’s software, the electron beam is scanned across the sample surface in a systematic raster pattern.
- Signal detection: Specialised detectors detect and collect secondary electrons and backscattered electrons generated from the sample surface.
- Image Formation: The detected signals are processed and turned into grayscale or colour images that depict the sample’s surface topography or compositional changes.
Magnification for Scanning Electron Microscopoy (SEM)
- SEM provides a wide range of magnification settings, from low to high.
- Adjusting the scanning settings and the distance between the sample and the detector controls the magnification.
- High-magnification imaging reveals intricate details of the sample’s surface by allowing visualisation of submicron and nanoscale structures.
Secondary Electron Detection
- Secondary electrons are low-energy electrons emitted from the sample surface as a result of the primary electron beam’s interaction.
- Secondary electrons are often collected using Everhart-Thornley detectors or scintillators.
- Secondary electron imaging gives topographic information by displaying the sample’s surface morphology and characteristics.
Backscattered Electron Detection
- Backscattered electrons are high-energy electrons that are elastically dispersed from the sample’s atomic nuclei.
- Backscattered electron detectors gather these electrons and quantify their intensity.
- Because the intensity of backscattered electrons is determined by the atomic number of the sample’s constituents, backscattered electron imaging offers compositional information.
Scanning Electron Microscopy Resolution
- SEM provides exceptional resolution, often ranging from sub-nanometer to a few nanometers.
- Factors such as electron beam energy, sample makeup, and detecting equipment utilised all influence resolution.
- SEM with high resolution allows for the visualisation of tiny surface features as well as the characterization of nanostructures.
Instrumentation:
- SEM generates an electron beam using an electron source, such as a tungsten filament or a field emission gun (FEG).
- The electron column is made up of lenses and scanning coils that focus and control the position and movement of the electron beam.
- SEM detectors include Everhart-Thornley detectors, scintillators, and energy-dispersive X-ray spectrometry (EDS) detectors.
- Vacuum System: To prevent electron scattering and preserve the integrity of the electron beam’s path, a high-vacuum environment is required.
Scanning Electron Microscopy Applications
- SEM is used in material science to analyse material surfaces, research crystal structures, and investigate material flaws.
- SEM allows for thorough imaging of biological samples such as cells, tissues, and organelles.
- SEM is used in nanotechnology to characterise nanomaterials such as nanoparticles, nanotubes, and nanowires.
- Geology and Earth Sciences: SEM is used to analyse minerals, rocks, and sedimentary structures, revealing information about their creation and composition.
- Forensics and Materials Failure Analysis: SEM is used in forensic investigations, failure analysis, and material defect or contamination assessment.
- Semiconductor Industry: SEM aids in the investigation of semiconductor materials, circuit inspection, and lithography process verification.
SEM has revolutionised the way we examine the microscopic world by delivering high-resolution images of surface topography and compositional information. SEM has evolved from humble origins to modern breakthroughs, becoming a vital instrument in a variety of domains such as materials research, life sciences, geology, and forensics. SEM continues to unravel the mysteries of the microscopic realm, opening new doors to scientific discoveries and technological developments, thanks to its extraordinary imaging capabilities and broad applications.